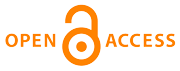 |
Home
>
Journal Issues
>
No 1 (2021) Technical mechanics
>
4
___________________________________________________
UDC 629.78
Technical mechanics, 2021, 1, 37 - 50
Problem of angular motion control of a non-cooperative on-orbit service object
DOI:
https://doi.org/10.15407/itm2021.01.037
Fokov A. A., Savchuk O. P.
Fokov A. A.
Institute of Technical Mechanics of the National Academy of Sciences of Ukraine and the State Space Agency of Ukraine
Savchuk O. P.
Institute of Technical Mechanics of the National Academy of Sciences of Ukraine and the State Space Agency of Ukraine
The realization of existing projects of on-orbit servicing and the development of new ones is a steady trend
in the development of space technology. In many cases, on-orbit service clients are objects that exhibit an
undesired rotary motion, which renders their servicing difficult or impossible. The problem of on-orbit
service object motion control determines the topicality of studies aimed not only at the refinement of
methods and algorithms of controlling both the translational and the rotary motion of an object, but also
at the development and refinement of methods of onboard determination of the object – service spacecraft
relative motion parameters. This paper overviews the state of the art of the problem of object motion
parameter determination in on-orbit servicing tasks and existing methods of object motion control and
angular motion damping and specifies lines of further investigations into the angular motion control
of non-cooperative service objects. Based on the analysis of publications on the subject, the applicability
of onboard means for object motion parameter determination is characterized. The analysis of the
applicability of methods of remote determination of the parameters of an unknown non-cooperative object
from a service spacecraft shows that they are at the research stage. The input data for the verification
of methods proposed in the literature were simulated or taken from ground experiments or previous missions.
Contact and contactless methods of angular motion control of non-cooperative on-orbit service objects are
considered. From the state of the art of investigations into the contactless motion control of on-orbit
service objects it may be concluded that the most advanced contactless method of motion control of an
on-orbit service object is a technology based on the use of an ion beam directed to the object from an
electrojet engine onboard a service spacecraft. Lines of further investigations into non-cooperative
object motion control are proposed.
non-cooperative on-orbit service object, onboard means of motion parameter determination, methods of angular motion control of objects,
overview of the state of the art of a problem, lines of investigations
1. Wei-Jie Li, Da-Yi Cheng, Xi-Gang Liu, Yao-Bing Wang, Wen-Hua Shi, Zi-Xin Tang, Feng Gao, Fu-Ming Zeng, Hong-You Chai, Wen-Bo Luo, Qiang Cong, Zhen-Liang Gao. On-orbit service (OOS) of spacecraft: A review of engineering developments. Progress in Aerospace Sciences. 2019. V. 108. Pp. 32-120.
https://doi.org/10.1016/j.paerosci.2019.01.004
2. Opromolla R., Fasano G., Rufino G., Grassi M. A review of cooperative and uncooperative spacecraft pose determination techniques for close-proximity operations. Progress in Aerospace Sciences. 2017. V. 93. Pp. 53-72
https://doi.org/10.1016/j.paerosci.2017.07.001
3. Fasano G., Accardo D., Grassi M. A Stereo-Vision Based System for Autonomous Navigation of an In-orbit Servicing Platform, 2009. ISBN-10: 1-56347-971-0, AIAA Infotech@Aero-space 2009, Seattle, USA. Pp. 1-10
4. D'Amico S., Benn M. Jorgensen J. L. Pose estimation of an uncooperative spacecraft from actual space imagery. International Journal of Space Science and Engineering. 2014. V. 2. No. 2. Pp. 171-189.
https://doi.org/10.1504/IJSPACESE.2014.060600
5. Naasz B. J., Burns R. D., Queen S. Z., Eepoel J. V., Hannah J., Skelton E. The HST SM4 relative navigation sensor system: overview and preliminary testing results from the flight robotics lab. The Journal of the Astronautical Sciences. 2009. V. 57, Nos. 1 & 2. Pp. 457-483.
https://doi.org/10.1007/BF03321512
6. Naasz B. J., Eepoel J. V., Queen S., Southward C., Hannah J. Flight results of the HST SM4 relative navigation sensor system. Proceedings in Advances in the Astronautical Sciences. 2010. V. 137. Pp. 723-744.
7. Liu C., Hu W. Relative pose estimation for cylinder-shaped spacecrafts using single image. IEEE Trans. Aerosp. Electron. Syst. 2014. No. 50. Pp. 3036-3056.
https://doi.org/10.1109/TAES.2014.120757
8. Du X., Liang B., Xu W., Qiu Y. Pose measurement of large non-cooperative satellite based on collaborative cameras. Acta Astronautica. 2011. V. 68. No. 11-12. Pp. 2047-2065.
https://doi.org/10.1016/j.actaastro.2010.10.021
9. Yu F., He Z., Qiao B., Vu X. Stereo-vision-based relative pose estimation for the rendezvous and docking of noncooperative satellites. Mathematical Problems in Engineering. 2014. Article ID 461283. 12 pp.
https://doi.org/10.1155/2014/461283
10. Blais F. Review of 20 Years of range sensor development. Journal of Electronic Imaging. 2004. V. 13. No. 1. Pp. 231-240.
https://doi.org/10.1117/1.1631921
11. Crosby S., Kang S. H. Object identification in 3D flash lidar images. Pattern Recognition. 2011. V. 6. No. 2. Pp. 193-200.
https://doi.org/10.13176/11.315
12. English C., Zhu S., Smith C., Ruel S., Christie I. Tridar: a hybrid sensor for exploiting the complementary nature of triangulation and LIDAR technologies. Proceedings of the 8th International Symposium on Artificial Intelligence, Robotics and Automation in Space. 2008. 9 pp.
13. Ruel S., English C., Anctil M., Church P. 3DLASSO: real-time pose estimation from 3D data for autonomous satellite servicing. Proceedings of the 2005 International Symposium on Artificial Intelligence for Robotics and Automation in Space. Munich, Germany. 2005. 8 pp.
14. Samson C., English C., Deslauriers A., Christie I., Blais F, Ferrie F. 3D Laser Camera System: from space mission STS-105 to terrestrial applications. Proceedings of the 2002 ASTRO Conference. Ottawa, Canada. Nov. 2002. 21 pp.
15. Liebe C. C., Abramovici A., Bartman R. K., Bunker R. L., Chapsky J., Chu C. C., Clouse D., Dillon J. W., Hausmann B., Hemmati H., Komfeld R. P., Kwa C., Mobasser S., Newell M., Padgett C., Roberts W. T., Spiers G., Warfield Z., Wright M. Laser radar for spacecraft guidance applications. Proceedings of 2003 IEEE Aerospace Conference. USA. 2003. V. 6. Pp. 2647-2662.
16. Kornfeld R. P., Bunker R. L, Cucullu G. C., Essmiller J. C., Hadaegh F. Y., Liebe C. C., Padgett C. W., Wong E. C. New millennium ST6 autonomous rendezvous experiment (ARX). Proceedings of the 2003 IEEE Aerospace Conference. USA. 2003. V. 1. Pp. 369-380.
17. Jenaoptronik. URL: https://www.jena-optronik.de/products/rendezvous-sensors/applications.html (Last accessed on March 11, 2021).
18. Smithpeter C. L., Nellums R. O., Lebien S. M., Studor G., James G. LADAR measurements of the international space station. Proceedings of SPIE Laser Radar Technology and Applications VI. 2001. V. 4377. Pp. 65-72.
https://doi.org/10.1117/12.440123
19. Advanced Scientific Concepts, Inc. URL: http://205.134.226.61/products/older-products/dragoneye.html (Last accessed on March 11, 2021).
20. Lauretta D. S. OSIRIS-REx asteroid sample-return mission. Handbook of Cosmic Hazards and Planetary Defense. 2015. Pp. 543-567.
https://doi.org/10.1007/978-3-319-03952-7_44
21. Advanced Scientific Concepts, Inc. URL: https://asc3d.com/product-overview (Last accessed on March 11, 2021).
22. Miller L. K., Masciarelli J., Rohrschneider R., Gravseth I. Critical advancement in telerobotic servicing vision technology. AIAA SPACE 2010 Conference & Exposition. 2010. Pp. 1-9.
https://doi.org/10.2514/6.2010-8919
23. Christian J. A., Patangan M., Hinkel H., Chevray K., Brazzel J. Comparison of orion vision navigation sensor performance from STD-134 and the space operations simulation center. AIAA Guidance, Navigation, and Control Conference. USA. 2012. Pp. 1-18.
https://doi.org/10.2514/6.2012-5035
24. Savchuk O. P., Fokov A. A. Non-cooperative object parameter determination in orbital service tasks. Teh. Meh. 2018. No. 4. Pp. 30-45. (in Russian).
https://doi.org/10.15407/itm2018.04.030
25. Doignon C., Stolkin R. An introduction to model-based pose estimation and 3-d tracking techniques. Scene Reconstruction, Pose Estimation and Tracking, first ed., InTech. 2007. Ch. 20. Pp. 359-382.
https://doi.org/10.5772/4943
26. Gubarev V. F., Boyun V. P., Melnichuk S. V., Salnikov N. N., Simakov V. A., Godunok L. A., Komosarenko V. I., Dobrovolskyy V. Yu., Derkach S. V., Matviyenko S. A. Using vision systems for determining the parameters of relative motion of spacecrafts. Journal of Automation and Information Sciences. 2016. V. 48. No. 11. Pp. 23-39.
https://doi.org/10.1615/JAutomatInfScien.v48.i11.30
27. Besl P., McKay N. A method for registration of 3-D shapes. IEEE Transactions on Pattern Analysis and Machine Intelligence. 1992. V. 14.No. 2. Pp. 239-256.
https://doi.org/10.1109/34.121791
28. Chen Y., Medioni G. Object modelling by registration of multiple range images. Image and Vision Computing. 1992. V. 10. No. 3. Pp. 145-155.
https://doi.org/10.1016/0262-8856(92)90066-C
29. Lichter M. D., Dubowsky S. State, shape, and parameter estimation of space objects from range images. Proceedings of the 2004 IEEE International Conference on Robotics & Automation. New Orleans, LA. 2004. Pp. 2974-2979.
https://doi.org/10.1109/ROBOT.2004.1307513
30. Pesce V., Lavagna M., Bevilacqua R. Stereovision-based pose and inertia estimation of unknown and uncooperative space objects. Advances in Space Research. 2017. V. 59. No. 1. Pp. 236-251.
https://doi.org/10.1016/j.asr.2016.10.002
31. Clohessy W. H., Wiltshire R. S. Terminal guidance system for satellite rendezvous. Journal of the Aerospace Sciences. 1960. V. 27. No. 9. Pp. 653-678.
https://doi.org/10.2514/8.8704
32. Gurfil P., Kholshevnikov K.V. Manifolds and metrics in the relative spacecraft motion problem. J. Guid. Control Dynamic. 2006. V. 29. No. 4. Pp. 1004-1010.
https://doi.org/10.2514/1.15531
33. Segal S., Gurfil P. Effect of kinematic rotation-translation coupling on relative spacecraft translational dynamics. J Guid Control Dynamic. 2009. V. 32. No. 3. Pp.1045-1050.
https://doi.org/10.2514/1.39320
34. Flores-Abad A., Ma O., Pham K., Ulrich S. A review of space robotics technologies for on-orbit servicing. Progress in Aerospace Sciences. 2014. V. 68. Pp. 1-26.
https://doi.org/10.1016/j.paerosci.2014.03.002
35. Nishida S., Kawamoto S. Strategy for capturing of a tumbling space debris. Acta Astronautica. 2011. V. 68. No. 1-2. Pp. 113 - 120.
https://doi.org/10.1016/j.actaastro.2010.06.045
36. CleanSpace One Gripper Report. Phase 0. Swiss Space Center EPFL. Lausanne, Switzerland, 2013. 67 pp.
37 Golovko M. G., Bezugly V. A., Bondarenko S. G., Rubakha Y. A., Pokrovskiy R. O. Technical aspects of space refuse control. Ecology and Noospherology. V. 23. No. 1-2. Pp. 110-120. (in Russian).
38. Space Transport Development Using Orbital Debris: Final Report on NIAC Phase I. Tether Applications, Inc.; J.A. Carroll. Research Grant No. 07600-087. 2002. 43 pp.
39. Clerc X., Retat I. Astrium Vision on Space Debris Removal. 63rd International Astronautical Congress. Naples, Italy. 2012. IAC-12.A6.7.4. 13 pp.
40. Fokov A. A., Savchuk O. P. Algorithms of the operation of a self-contained system for slowing down the rotation of a non-cooperative object of on-orbit servicing. Teh. Meh. 2019. No. 4. Pp. 44-58. (in Russian).
https://doi.org/10.15407/itm2019.04.044
41. Chang H., Huang P., Lu Z., Meng Z., Liu Z., Zhang Y. Cellular space robot and its interactive model Identification for spacecraft takeover control. International Conference on Intelligent Robots and Systems (IROS). Daejeon, Korea. 2016. Pp. 3069-3074.
https://doi.org/10.1109/IROS.2016.7759475
42. Aslanov V. S., Ledkov A. S. Attitude motion of cylindrical space debris during its removal by ion beam. Mathematical Problems in Engineering. 2017. V. 2017. Article ID 1986374. 7 pp.
https://doi.org/10.1155/2017/1986374
43. Aslanov V. S., Ledkov A. S. Chaotic motion of a passive space object during its contactless transportation by ion beam. 2020 International Conference on Information Technology and Nanotechnology (ITNT). Samara, Russia: IEEE, 2020. Pp. 1-6.
https://doi.org/10.1109/ITNT49337.2020.9253185
44. Aslanov V. S., Ledkov A. S. Space debris attitude control during contactless transportation in planar case. Journal of Guidance, Control, and Dynamics. 2020. V. 43. No. 3. Pp.451-461.
https://doi.org/10.2514/1.G004686
45. Aslanov V., Ledkov A., Konstantinov M. Chaotic motion of a cylindrical body during contactless transportation from MEO to LEO by ion beam. Nonlinear Dynamics. 2020. V. 101. No. 2. Pp. 1221-1231.
https://doi.org/10.1007/s11071-020-05822-0
46. Aslanov V. S., Ledkov A. S. Dynamics and control of space debris during its contactless ion beam assisted removal. Journal of Physics: Conference Series. 2020. V. 1705. Paper 012006. Pp. 1-10.
https://doi.org/10.1088/1742-6596/1705/1/012006
47. Alpatov A. P., Khoroshylov S. V., Maslova A. I. Contactless De-Orbiting of Space Debris by the Ion Beam. Êyiv: Akademperiodyka, 2019. 70 pp.
https://doi.org/10.15407/akademperiodyka.383.170
48. Stevenson D., Schaub H. Multi-sphere method for modeling electrostatic forces and torques. Advances in Space Research. . 2013. V. 51. No. 1. Pp. 10-20.
https://doi.org/10.1016/j.asr.2012.08.014
49. Schaub H., Sternovsky Z. Active space debris charging for contactless electrostatic disposal maneuvers. Advances in Space Research. 2014. V. 53. No. 1. Pp. 110-118.
https://doi.org/10.1016/j.asr.2013.10.003
50. Stevenson D. Remote Spacecraft Attitude Control by Coulomb Charging. Ph.D. Dissertation. Aerospace Engineering Sciences Dept. Univ. of Colorado, Boulder, CO. May 2015. 122 pp.
51. Schaub H., Stevenson D. Electrostatic spacecraft rate and attitude control-Experimental results and performance considerations. Acta Astronautica. 2016. V. 119. P. 22-33.
https://doi.org/10.1016/j.actaastro.2015.10.023
52. Bengtson M., Wilson K., Hughes J., Schaub H. Survey of the electrostatic tractor research for reorbiting passive GEO space objects. Astrodynamics. 2018. V. 2. No. 4. Pp. 291-305.
https://doi.org/10.1007/s42064-018-0030-0
53. Aslanov V. S., Dynamics and control of an active satellite in space debris towing with the use of Coulomb forces. Proceedings of the XIIth All-Russian Congress on Fundamental Problems in Theoretical and Applied Mechanics. Ufa, August 19-24, 2019. Ufa: Bashkir State University. 2019. Pp. 38-39 (in Russian).
54. Aslanov V., Schaub H. Detumbling attitude control analysis considering an electrostatic pusher configuration. Journal of Guidance, Control, and Dynamics. 2019. V. 42. No. 4. Pp. 900-909.
https://doi.org/10.2514/1.G003966
55. Khoroshylov S. V. The algorithm to control the in-plane relative motion of a spacecraft for contactless space debris removal. Space Sci. & Technol. 2019. V. 25. No. 1. Pp. 14-26. (in Russian).
https://doi.org/10.15407/scine14.04.005
56. Khoroshylov S. Out-of-plane relative control of an ion beam shepherd satellite using yaw attitude deviations Acta Astronautica. 2019. V. 164. Pp. 254-261.
https://doi.org/10.1016/j.actaastro.2019.08.016
57. Alpatov A. P., Fokov A. A., Khoroshylov S. V., Savchuk A. P. Error analysis of method for calculation of non-contact impact on space debris from ion thruster. Mechanics, Materials Science & Engineering. 2016. No. 5. Pp. 64-76.
Copyright (©) 2021 Fokov A. A., Savchuk O. P.
Copyright © 2014-2021 Technical mechanics
____________________________________________________________________________________________________________________________
|
GUIDE FOR AUTHORS
====================
Open Access Policy
====================
REGULATIONS
on the ethics of publications
====================
|