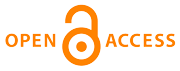 |
Home
>
Journal Issues
>
No 1 (2022) Technical mechanics
>
1
___________________________________________________
UDC 629.76
Technical mechanics, 2022, 1, 3 - 15
Assessment of thrust chamber stability margins to high-frequency oscillations based on mathematical modeling of coupled ‘injector – rocket combustion chamber’ dynamic system
DOI:
https://doi.org/10.15407/itm2022.01.003
Nikolayev O. D., Bashliy I. D
Nikolayev O. D.
Institute of Technical Mechanics of the National Academy of Sciences of Ukraine and the State Space Agency of Ukraine
Bashliy I. D
Institute of Technical Mechanics of the National Academy of Sciences of Ukraine and the State Space Agency of Ukraine
High-frequency instability of a liquid-propellant rocket engine (LRE) during static firing tests is often
accompanied by a significant increase in dynamic loads on the combustion chamber structure, often leading
to a chamber destruction. This dynamic phenomenon can also be extremely dangerous for the dynamic strength
of a liquid-propellant rocket engine. The calculation of acoustic combustion product oscillation parameters
is important in the design and static firing tests of such rocket engines. The determination of the oscillation
parameters (natural frequencies and stability margins such as oscillation decrement) is one of the problems
solved in the LRE design period as part of the development of measures to ensure the engine stability.
The main aim of the paper is to develop a numerical approach to determining the parameters of acoustic oscillations
of combustion products in liquid-propellant rocket engines combustion chambers taking into account the features of
combustion space configuration and the variability of gaseous medium physical properties depending on the axial
length of the chamber, acoustic impedance in critical throat and dissipation effects (damping experimental values)
in the shell structure and the gas media in the chamber. The approach is based on mathematical modeling of the
coupled ‘chamber shell structure – gas’ dynamic system by using the finite element method and the CAE (Computer
Aided Engineering) system.
The developed approach testing and further analysis of the results for the RD 253 engine using nitrogen tetroxide
and unsymmetrical dimethylhydrazine as a propellant pair were carried out. The dynamic system shapes and frequencies
of longitudinal, tangential and radial modes are determined. The results of mathematical modeling of the dynamic
system indicate a satisfactory agreement of the calculated decrements of the first longitudinal oscillation mode
and third tangential oscillation mode with the experimental decrements obtained by hot-fire tests data. From system
harmonic analysis of the thrust chamber, it follows that the dynamic pressure gain factor of the gas media in the
chamber at the first longitudinal mode frequency is 1.6 times greater than the system dynamic gain in the tangential
mode. At the same time, the oscillation decrement for the system tangential mode is 2 times smaller than that of the
first longitudinal mode. This means that the thrust chamber tangential mode is more dangerous and can lead to rocket
engine combustion instability.
The effect of the injector on the high-frequency stability of the combustion chamber and the possibility of partial
suppression of combustion chamber thermoacoustic oscillations by adjusting the high-frequency dynamics of the
injector are shown theoretically.
liquid-propellant rocket engine, combustion chamber, high-frequency instability, oscillation frequencies, logarithmic decrements (oscillation decrements)
1. Natanzon M. C. Combustion Instability. Moscow, 1986. 208 pp. (in Russian).
2. Dranovsky M. L. Combustion instabilities in liquid rocket engines. testing and development practices in Russia, AIAA Progress In Astronautics And Aeronautics. 2007. V. 221. Ð. 321.
https://doi.org/10.2514/4.866906
3. Ylchenko M. A., Kriutchenko V. V., Mnatsakanian Yu. S. Operation Process Stability in Flying Vehicle Engines. Ìoscow, 1995. 320 pp. (in Russian).
4. Klein S., Borner M., Hardi J. S., Suslov D., Oschwald M. Injector coupled thermoacoustic instabilities in an experimental LOX methane rocket combustor during start up. CEAS Space Journal. 2020. V. 12. No. 2. Ðp. 267-279.
https://doi.org/10.1007/s12567-019-00294-4
5. Oschwald M., Farago Z., Searby G., Cheuret F. Resonance frequencies and damping of a combustor acoustically coupled to an absorber. Journal of Propulsion and Power. 2008. V. 24. No. 3. Ðp. 524-533.
https://doi.org/10.2514/1.32313
6. Mosolov S. V., Sydlerov D. A. Impact research of pulsation control baffles on development of operation process in combustion chamber of oxygen-kerosene LRE with jet-centrifugal injectors by method of numerical simulation. Vestnyk MHTU im. N .E. Baumana. Seryia Mashynostroenye. 2017. No. 2(113). URL: https://cyberleninka.ru/article /n/issledovanie-vliyaniya-antipulsatsionnyh-peregorodok-na-razvitie-rabochego-protsessa-v-kamere-sgoraniya-kislorodno-kerosinovogo-zhrd-s (Last accessed on December 10, 2019). (in Russian).
7. Kalmykov G. P., Larionov A. A., Sidlerov D. A., Yanchilin L. A. Numerical simulation and investigation of working process features in high-duty combustion chambers. Journal of Engineering Thermophysics. 2008. V. 17. No. 3. Pp. 196-217. (in Russian).
https://doi.org/10.1134/S1810232808030053
8. Crocco L., Cheng S.I. Theory of Combustion Instability in Liquid Propellant Rocket Motors. London: Butterworths Scientific Publications; New York: Interscience Publishers Inc., 1956. 200 pp.
9. Kaess R., Koeglmeier S., Sattelmayer T., Schulze M., Oschwald M., Hardi J. HF Combustion Stability. Research Activities in Germany, Space Propulsion Conference. Rome. 2016. 12 pp., SP2016_3124816. URL: https://elib.dlr.de/107846/1/Kaess2016_SP2016_3124816.pdf (Last accessed on October 25, 2020).
10. Gerhold T., Friedrich O., Evans J., Galle M. Calculation of Complex Three-Dimensional Configurations Employing the DLR-TAU-Code. 1997. AIAA. Paper 97-0167.
https://doi.org/10.2514/6.1997-167
11. Marco L., Francesco N., Marcello O. Non-linear analysis of low-frequency combustion instabilities in liquid rocket engines. Progress in Propulsion Physics. 2019. V. 11. Ðp. 295-316.
12. Pylypenko V. V., Zadontsev V. A., Natanzon M. S. Cavitation Self-Exñited Oscillations and Dynamics of Hydraulic Systems. Moscow: Mashinostroenie, 1977. 352 ðp. (in Russian).
13. Kohnke P. Ansys Inc. Theory Manual. Twelfth Edition, Canonsburg, 2001. 1266 pp.
14. Zenkevych O. K. Finite-Element Method in Engineering. Moscow, 1975. 541 ðp. (in Russian).
15. Lependyn L. F. Acoustics. Moscow, 1978. 448 ðp. (in Russian).
16. Rouch P. Computational Fluid Dynamics. M., 1980. 618 ðp. (in Russian).
17. Bate K. Yu. Finite-Element Methods. Moscow, 2010. 1024 ðp. (in Russian).
18. Zienkiewicz O. C., Newton R. E. Coupled Vibrations of a structure submerged in a compressible fluid. Proceedings of the Symposium on Finite Element Techniques. University of Stuttgart, Germany. June, 1969. URL: https://repository.tudelft.nl/islandora/object/uuid%3A27785b4f-3709-4fa9-a189-6ce1d3365564 (Last accessed on October 25. 2020).
19. Lebedynskyi E. V., Natanzon M. S., Nykyforov M. V. Experimental method for calculating the dynamic properties of gas flows. Acoustic Journal. 1982. V. XXVIII. No. 5. Ðp. 660-664. (in Russian).
20. Chelomei V. N., Henkyn M. D. Vibrations in Engineering. Measurements and Tests. Moscow: Mashynostroyenye, 1981. V. 5. 496 pp. (in Russian).
Copyright (©) 2022 Nikolayev O. D., Bashliy I. D
Copyright © 2014-2022 Technical mechanics
____________________________________________________________________________________________________________________________
|
GUIDE FOR AUTHORS
====================
Open Access Policy
====================
REGULATIONS
on the ethics of publications
====================
|