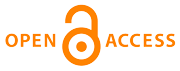 |
Home
>
Journal Issues
>
No 3 (2021) Technical mechanics
>
2
___________________________________________________
UDC 532.528:621
Technical mechanics, 2021, 3, 9 - 22
CURRENT PROBLEMS IN THE LOW-FREQUENCY DYNAMICS OF LIQUID-PROPELLANT ROCKET PROPULSION SYSTEMS
DOI:
https://doi.org/10.15407/itm2021.03.009
Pylypenko O. V., Nikolayev O. D., Khoriak N. V., Dolgopolov S. I., Bashliy I. D
Pylypenko O. V.
Institute of Technical Mechanics of the National Academy of Sciences of Ukraine and the State Space Agency of Ukraine
Nikolayev O. D.
Institute of Technical Mechanics of the National Academy of Sciences of Ukraine and the State Space Agency of Ukraine
Khoriak N. V.
Institute of Technical Mechanics of the National Academy of Sciences of Ukraine and the State Space Agency of Ukraine
Dolgopolov S. I.
Institute of Technical Mechanics of the National Academy of Sciences of Ukraine and the State Space Agency of Ukraine
Bashliy I. D
Institute of Technical Mechanics of the National Academy of Sciences of Ukraine and the State Space Agency of Ukraine
One of the key problems in liquid-propellant rocket engine (LPRE) design is to provide the stability of
LPRE working processes, in particular low-frequency stability. In LPRE experimental tryout, every so
often there occur situations where the development of divergent oscillations set up in some of the
LPRE loops or units results in contingencies: exceeding the engine ultimate strength, pump stall,
chamber ignition, etc. Such contingencies may lead to grave consequences, including engine and bench
equipment failure. Because of this, mathematical simulation is one of the main tools that allow one to
predict he dynamic performance of an LPRE both in its steady operation and in transients and its
startup operation features at the design and tryout stage. This paper overviews and analyzes scientific
publications for the past 15 years concerned with the study of the dynamics and low-frequency stability
of advanced LPREs and units thereof along different lines. This analysis made it possible to identify
problems in low-frequency stability prediction and assurance for liquid-propellant rocket propulsion
systems (LPRPSs) under design, to cover new research results (experimental and theoretical) on the
origination and development of all-engine low-frequency oscillations and low-frequency oscillations
in LPRPS systems and units and to identify new approaches to the mathematical simulation and study
of low-frequency processes in LPRPSs and promising lines of investigation. The man lineû of the
analysis are as follows: the low-frequency dynamics of cavitating inducer-equipped centrifugal pumps
and LPRE gas paths, LPRE thrust control problems, the interaction of launch vehicle airframe
longitudinal oscillations with low-frequency processes in the sustainer LPRPS, dynamic processes
during an LPRE startup/shutdown, and low-frequency in-chamber oscillations.
liquid-propellant rocket engine, low-frequency stability, all-engine oscillations, cavitation,
inducer-equipped centrifugal pump, feed system, gas generator, combustion chamber
1. Cervone A., Torre L., Pasini A., d'Agostino L. Cavitation and turbopump hydrodynamics research at Alta S. P. A. and Pisa University. Proc. of the 4 th Int. Symposium on Fluid Machinery and Fluid Mechanics, Beijing (China). 2008. No. 4ISFMFE-IL16. 8 pp.
2. Ehrlich D., Schwille J., Welle R., Murdock J., Hardy B. water test facility for liquid rocket engine turbopump cavitation testing. Proc. of the 7-th Int. Symposium on Cavitation, Ann Arbor, Michigan (USA). 2009. CAV2009, Paper No. 11. 10 pp.
3. Zadontsev V. A., Drozd V. A., Dolgopolov S. I., Grabovskaya T. A. Off-engine dynamic tests of a large-sized LPRE inducer-equipped centrifugal pump under cavitation self-oscillations. Aviatsionno-Kosmicheskaya Tekhnika i Tekhnologiya. 2009. No. 9(66). Pp. 100-106. (in Russian).
4. Dinardi Aaron, Capozzoli Peter, Shotwell Gwynne. Low-cost launch opportunities provided by the Falcon family of launch vehicles. The Fourth Asian Space Conference, 2008, Taipei, Taiwan, 1-3 October 2008. https://selenianboondocks.com/wp-content/uploads/2008/11/s12-11.pdf (last accessed on February 22, 20201).
5. Cervone Angelo, Torre Lucio, Bramanti Cristina, Rapposelli Emilio, d'Agostino Luca. Experimental characterization of cavitation instabilities in the Avio "FAST2" inducer. AIAA/ASME/SAE/ASEE Joint Propulsion Conference: 41st Conference & Exhibit, 10 July 2005 - 13 July 2005, Tucson, Arizona. https://doi.org/10.2514/6.2005-4451. Published online on 19 Jun. 2012.
https://doi.org/10.2514/6.2005-4451
6. Arnone A., Boncinelli P., Capuani A., Spano E., Rebattet C. "ARIANE 5" tpLOX inducer design strategies to enhance cavitating performance. CAV 2001: 4th International Symposium on Cavitation, June 20-23, 2001, California Institute of Technology, Pasadena, CA USA. Conference paper CAV2001:sessionB7.004. 12 pp. URL: https://resolver.caltech.edu/CAV2001: sessionB7.004 (last accessed on March 23, 20201).
7. Christopher E. Brennen. Multifrequency instability of cavitating inducers. Journal of Fluid Engineering. 2007. Vol. 129(6). Pp. 731-736.
https://doi.org/10.2514/6.2005-4451
8. Hadavandi Ruzbeh, Pasini Angelo, Valentini Dario, Pace Giovanni, d'Agostino Luca. Thermal cavitation instability analysis in axial inducers by means of casing and hub-mounted pressure sensors. Proceedings of the ASME-JSME-KSME, 2019. Joint Fluids Engineering Conference AJKFLUIDS2019 (July 28 -Aug. 1, 2019), San Francisco, CA, USA. Joint Fluids Engineering Conference AJKFLUIDS2019 (July 28 - Aug. 1, 2019), San Francisco, CA, USA. Published Online: November 20, 2019. Paper No: AJKFluids2019-5620, V03BT03A04.; 12 pp. https://doi.org/10.1115/AJKFluids2019-5620
https://doi.org/10.1115/AJKFluids2019-5620
9. Christopher E. Brennen. On the dynamics of a cavitating pump. 28th IAHR Symposium on Hydraulic Machinery and Systems (IAHR2016) IOP. Publishing IOP Conf. Series: Earth and Environmental Science 49(5): 052018. 10 pp.
https://doi.org/10.1088/1755-1315/49/5/052018
10. Yoshida Yo., Nanri H., Kikuta K., Kazami Yu., Iga Yu., Ikohage T. Thermodynamic effect on subsynchronous rotating cavitation and surge mode oscillation in a space inducer. ASME J. Fluid Eng. June 2011. V. 133 (6). 061301. P. 7.
https://doi.org/10.1115/1.4004022
11. Koshelev I. M., Martirosov D. S., Kolbasenkov A. I. Effect of high-temperature oxidizing gas condensation in the cryogen oxygen zone on LPRE low-frequency stability. Dvigatel. 2012. No. 6(84). Pp. 24-27. (in Russian).
12. Turnov M. A. Experience of the bench tryout of liquid-oxygen feed system components for the Energiya launch vehicle. Polet. 2009. No. 10. Pp. 35-40. (in Russian).
13. Katkov R. E., Kiseleva O. V., Strizhenko P. P., Tupitsyn N. N. Experimental study of alternate designs for a jet condenser pump a part of a LOX booster turbopump assembly. Kosmicheskaya Tekhnika i Tekhnologiya. . 2017. No. 1(16). Pp. 63-70. (inj Russian).
14. Biryukov V. I., Tsarapkin A. R. Stability margin estimation technique for the working process in liquid-propellant rocket engine combustion chambers and gas generators. News of the Tula State University. Technical Sciences. 2017. No. 5. Pp. 19-33. (in Russian).
15. Dorosh N. L. Simulation of the condensation of an oxygen vapor jet in liquid oxygen. Applied Questions of Mathematical Modeling. 2020. V. 3. No. 2.2. Pp. 149-155. (in Ukrainian).
https://doi.org/10.32782/KNTU2618-0340/2020.3.2-2.14
16. Belyaev E. N., Kolomentsev A. I., Nasimento L. B., Nazarov V. P. Evvect of the design parameters of a flow controller on its static and dynamic performance. Vestnik of SibGAU. 2014. No. 1 (53). Pp. 109-113. (in Russian).
17. Dolgopolov S. I., Nikolaev A. D. Mathematical modelling low-frequency dynamics of flow controller at various amplitudes of harmonic disturbance. Teh. Meh. 2017. No. 1. Pp. 3-13. (in Russian).
https://doi.org/10.15407/itm2017.01.015
18. Ryan Lundgreen. Water Turbopump Inducer Performance with and without a Stability Control Device. 2013. P. 6. URL: https://digitalcommons.usu.edu/cgi/viewcontent.cgi?article=1004&context=spacegrant (last accessed on January 28, 20201).
19. Perez-Roca Sergio, Marzat Julien, Flayac Emilien, Piet-Lahanier Helene, Langlois Nicolas, Farago Francois, Galeotta Marco, Le Gonidec Serge. An MPC approach to transient control of liquid-propellant rocket engines. Science Direct. IFAC-PapersOnLine. 2019 (Nov.). V. 52. Iss. 12. Pp. 268-273.
https://doi.org/10.1016/j.ifacol.2019.11.254
20. Casiano Matthew J. Hulka James R., Yang Vigor. Liquid-propellant rocket engine throttling: A comprehensive review. Journal of Propulsion and Power. 2010. V. 26. No. 5. Pp. 897-923.
https://doi.org/10.2514/1.49791
21. Gemranova E. A., Kolbasenkov A. I., Koshelev I. M., levochkin P. S., Martirosov D. S. Methods to suppress low-frequency oscillations in a deeply throttled LPRE. Proceedings of NPO Energomash. 2013. No 30. Pp. 104-110. (in Russian).
22. Pylypenko O. V., Prokopchyuk A. A., Dolgopolov S. I., Khoriak N. V., Nikolaev A. D., Pisarenko V. Yu., Kovalenko V. N. Mathematical simulation and stability analysis of low-frequency processes in staged-combustion sustainer LPRE. Vestnik Dvigatelestroyeniya. 2017. No. 2. Pp. 34-42. (in Russian).
23. Khoriak N. S., Nikolaev A. D. Decomposition and stability analysis of a dynamic system "feed pipelines - oxidized-rich staged-combustion sustainer LPRE". Teh. Meh. 2007. No. 1. Pp. 28-42. (in Russian).
24. Khoriak N. V., Dolhopolov S. I. Features of mathematical simulation of gas path dynamics in the problem of the stability of low-frequency processes in liquid-propellant rocket engines. Teh. Meh. 2017. No. 3. Pp. 30-44. (in Russian).
https://doi.org/10.15407/itm2017.03.030
25. Pylypenko O. V., Dolhopolov S. I., Nikolayev O. D., Khoriak N. V. Mathematical simulation of the start of a multiengine liquid-propellant rocket propulsion system. Teh. Meh. 2020. No. 1. Pp. 5-19. (in Russian).
https://doi.org/10.15407/itm2020.01.005
26. Belyaev E. N., Vorob'ev A. G. Effect of the filling of gas generator mixing heads on the starterless startup dynamics of a liquid-propellant rocket engine. Sibirskii Zhurnal Nauki i Tekhnologii. 2018. V. 19. No. 3. Pp. 469-481. (in Russian).
https://doi.org/10.31772/2587-6066-2018-19-3-469-481
27. Belyaev E. N., Vorob'ev A. G., Gnesin E. M. Development of a nonlinear mathematical model for the steady-operation of a liquid-propellant rocket engine. Trudy MAI. 2013. No. 73. 18 pp. URL: https://mai.ru/upload/iblock/346/346116506f3672611cd1833c7a2b319f.pdf (last accessed on February 12, 2021). (in Russian).
28. Chen Liping, Xie Gang, Ding Ji, Zhang Haiming, Yang Hao. Modeling and simulation of liquid propellant rocket engine transient performance using Modelica. Proc. of the 11th International Modelica Conference (Sept. 21-23, 2015). Versailles, France, 2015. Ðp. 485-490. URL: https://ep.liu.se/ecp/118/052/ecp15118485.pdf (last accessed on February 25, 2021).
29. Pylypenko O. V., Khoriak N. V., Dolhopolov S. I., Nikolayev O. D. Mathematical simulation of dynamic processes in hydraulic and gas paths at the start of a liquid-propellant rocket engine with generator gas after-burning. Teh. Meh. 2019. No. 4. Pp. 5-20. (in Russian)
https://doi.org/10.15407/itm2019.04.005
30. Sherstyannikov V. N. Study of a dynamic liquid-propellant multichamber propulsion system. Dvigatel. 2003, No. 4 (28). P. 35. http://engine.aviaport.ru/issues/28/page35.html. (in Russian).
31. Marco Leonardi, Francesco Di Matteo, Johan Steelant, Francesco Nasuti, Marcello Onofri. Non-linear analysis of low-frequency combustion instabilities in liquid rocket engines. 6th European Conference for Aeronautics and Space Sciences (Eucass). Conference Paper. June 2015. URL: https://doi.org/10.1051/eucass/201911295 (last accessed on January 18, 2021).
https://doi.org/10.1051/eucass/201911295
32. Oppenheim B. W., Rubin S. Advanced pogo stability analysis for liquid rockets. Journal of Spacecraft and Rockets. 1993. V. 30. No. 3. Pp. 360-383.
https://doi.org/10.2514/3.25524
33. Pylypenko V. V., Dovgotko N. I., Nikolaev A. D., Dolgopolov S. I., Khoriak N. V., Sirenko V. A. Theoretical determination of active-flight dynamic loads (longitudinal vibration accelerations) on the RS-20 liquid-propellant rocket structure. Teh. Meh. 2000. No. 1. Pp. 3-18. (in Russian).
34. Chimeh S.A.J., Shahi H.K.M., Dehaj M.S. Frequency Analysis of launch vehicle oscillation absorbent configuration on performance of liquid propellant engine. Journal of Aeronautics and Space Technologies. 2019. V. 12. No. 1. Ðp. 109-120.
35. Pylypenko O.V., Degtyarev M. A., Nikolayev O. D., Klimenko D. V., Dolgopolov S. I., Khoriak N. V., Bashliy I. D., Silkin L. A. Providing of POGO stability of the Cyclone-4M launch vehicle. Space Science and Technology. 2020. V. 26. No. 4. Pp. 3 - 20.
https://doi.org/10.15407/knit2020.04.003
36. Filippovsky S. V., Avramov K. V. Finite element analysis of resonant modes of fuel injection tract missiles. Bulletin of the National Technical University "KhPI". Series: Dynamics and Strength of Machines. 2009. No. 30. Pp.183-190. (in Russian).
37. Khoriak N. V., Nikolaev A. D. Mathematical simulation of interaction between longitudinal vibrations of the airframe of a liquid-propellant rocket as a multiply connected elastodissipative system and dynamic processes in the propulsion system. Teh. Meh. 2010. No. 3. Pp. 27-37. (in Russian).
38. Kook Jin Park, JeongUk Yoo, SiHun Lee, Jaehyun Nam, Hyunji Kim Juyeon Lee, Tae-Seong Roh, Jack J. Yoh, Chongam Kim, SangJoon Shin. Pogo accumulator optimization based on multiphysics of liquid rockets and neural networks. Journal of Spacecraft and Rockets. 2020. V. 57. No. 4. Ðp. 809-822.
https://doi.org/10.2514/1.A34769
39. Qingwei Wang, Shujun Tan, Zhigang Wu, Yunfei Yang, Ziwen Yu. Improved modelling method of Pogo analysis and simulation for liquid rockets. Acta Astronautica. 2015. V. 107. Ðp. 262-273.
https://doi.org/10.1016/j.actaastro.2014.11.034
40. Nikolayev O. D., Bashliy I. D., Khoriak N. V. Ñomputation of the POGO self-oscillation parameters in dynamic "propulsion - rocket structure" system by using a 3D structural model. Teh. Meh. 2018. No. 2. Pp. 17 - 29.
https://doi.org/10.15407/itm2018.02.017
Copyright (©) 2021 Pylypenko O. V., Nikolayev O. D., Khoriak N. V., Dolgopolov S. I., Bashliy I. D
Copyright © 2014-2021 Technical mechanics
____________________________________________________________________________________________________________________________
|
GUIDE FOR AUTHORS
====================
Open Access Policy
====================
REGULATIONS
on the ethics of publications
====================
|