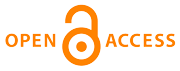 |
Home
>
Journal Issues
>
No 1 (2020) Technical mechanics
>
5
___________________________________________________
UDC 629.78
Technical mechanics, 2020, 1, 56 - 66
FEATURES OF THE USE OF MAGNETIC CONTROLS IN A COARSE STABILIZATION OF SPACECRAFT WITH AEROMAGNETIC DEORBIT SYSTEMS
DOI:
https://doi.org/10.15407/itm2020.01.056
Lapkhanov E. O.
Lapkhanov E. O.
Institute of Technical Mechanics of the National Academy of Sciences of Ukraine and the State Space Agency of Ukraine
The development of hybrid deorbit means for used spacecraft is a promising line in the elaboration of space
debris mitigation technologies. The main objective of this line is a search for optimal solutions in the
development of new means for spacecraft removal from near-Earth orbits with account for certain operating
limitations on the use of existing deorbit systems. So the advantage of hybrid deorbit means lies in
broadening the scope of application of modern deorbit systems by combining certain technical features
of each of them when developing a new system.
One of the lines in the development of hybrid means for space debris deorbit is the development of
aeromagnetic deorbit systems for removing used spacecraft from low-Earth orbits. This class of systems
features the possibility of controlled deorbit when using aerodynamic flat sailing elements. The control
objective is the angular stabilization of a flat aerodynamic element perpendicular to the incident
atmospheric flow. . Studies have shown that this stabilization of a flat sailing element increases the
aerodynamic drag by 20–40 % and reduces the deorbit time by 25–30 % as compared to nonoriented deorbit,
which broadens the scope of application of aerodynamic sailing deorbit systems. In aeromagnetic deorbit
systems, the control actuators are magnetic attitude control systems (MACSs). The main criterion for the
MACS effectiveness in a particular mission is a minimum of onboard power consumption. This may be achieved
by using permanent-magnet actuators or spacecraft electromagnets (magnetorquers) in the rough stabilization
mode. In its turn, in the rough stabilization mode the onboard power consumption is minimized when using
time-shared control methods for the magnetorquers and a nonlinear discrete control law for the
permanent-magnet actuators.
The aim of this paper is to develop methodological foundations for the use of permanent-magnet actuators
and magnetorquers in the attitude control of used spacecraft with aeromagnetic deorbit systems. The paper
makes an analytical comparison of the use of permanent-magnet actuators and magnetorquers depending on the
spacecraft design features, mass, size, and energy characteristics and presents an algorithm of MACS
choice for spacecraft of various classes equipped with aeromagnetic deorbit systems.
aeromagnetic deorbit system, magnetic attitude control systems, spacecraft, deorbit
1. The Orbital Debris Quarterly News. NASA JSC Houston. 2019. Iss. 4. V. 23. P. 10.
2. Alpatov A. P., Goldstein Yu. M. Ballistic analysis of orbits distribution of spacecraft for different functional missions. Teh. Meh. 2017. No. 2. Pp. 33-40. (in Russian)
https://doi.org/10.15407/itm2017.02.033
3. Alpatov A. P., Holdshtein Yu. M. On the choice of the ballistic parameters of an on-orbit service spacecraft. Teh. Meh. 2019. No. 1. Pp. 25- 37.
https://doi.org/10.15407/itm2019.01.025
4. Kessler D. J., Johnson N. L., Liou J.-C., Matney M. The Kessler syndrome: Implications to future space operations. 33rd Annual AAS Gguidance and Control Conference. Breckenridge, Colorado. February 6-10, 2010. URL: http://citeseerx.ist.psu.edu/viewdoc/download?doi=10.1.1.394.6767&rep =rep1&type=pdf (last accessed Jan. 25, 2020).
5. Minin A., Afanas'ev I. The start of the Starlink mission - Internet for the whole of the world. Russkii Kosmos. 2019. Pp. 42-45. URL: https://www.roscosmos.ru/media/img/2019/august/rk2019-07.pdf (last accessed Jan. 25, 2020). (in Russian).
6. Alpatov A. P. Information methods and technologies to control the anthropogenic pollution of near space. System Technologies. 2018. No. 3 (116). Pp. 3-14. (in Russian).
7. Alpatov A. P., Maslova A. I., Khoroshylov S. V. Contactless Removal of Space Debris with an Ion Beam. International Book Market Sevice Ltd, member of OmniScriptum Publishing Group, Beau Bassin. 2018. 331 pp. (in Russian).
8. Shan M., Guo J., Gill E. Review and comparison of active space debris capturing and removal methods. Pro-gress in Aerospace Sciences. 2016. V. 80. Pp. 18-32.
https://doi.org/10.1016/j.paerosci.2015.11.001
9. Pelton J. N. New Solutions for the Space Debris Problem. Springer. 2015. 94 pp.
https://doi.org/10.1007/978-3-319-17151-7
10. Lapkhanov E. O. Features of the development of means for spacecraft removal from near-Earth operational orbits. Teh. Meh. 2019. No. 2. Pp. 16-30. (in Ukrainian).
https://doi.org/10.15407/itm2019.02.016
11. Pikalov R. S., Yudintsev V. V. Overview and choice of means for the removal of large-size space debris. Trudy MAI. 2018. No. 100. URL: http://trudymai.ru/upload/iblock/239/Pikalov_YUdintsev_rus .pdf?lang=ru&issue=100 (last accessed Jan. 25, 2020). (in Russian).
12. Paliy A. S. Methods and means for spacecraft deorbit (state of the art). Teh. Meh. 2012. No. 1. Pp. 94-102. (in Russian).
13. Khoroshilov S. V. Synthesis of robust controller for ion beam shepherd control system. Teh. Meh. 2017. No. 1. Pp. 26-39. (in Russian).
https://doi.org/10.15407/itm2017.01.026
14. Dron' M., Golubek O., Dubovik L., Dreus A., Heti K. Analysis of the ballistic aspects of the combined method of deorbiting space objects from the near-Earth orbits. Eastern-European Journal of Enterprise Technologies. 2019. No. 2/5 (98). Pp. 49-54.
https://doi.org/10.15587/1729-4061.2019.161778
15. Svorobin D. S., Fokov A. A., Khoroshylov S. V. Analysis of using an aerodynamic compensator in contactless space debris removal. Aviatsionno-Kosmicheskaya Tekhnika i Tekhnologiya. 2018. No. 6. Pp. 4-11. (in Russian).
16. Lapkhanov E. Khoroshylov S. Development of the aeromagnetic space debris deorbiting system. Eastern-European Journal of Enterprise Technologies. 2019. V. 5. No. 5(101). Pp. 30-37.
https://doi.org/10.15587/1729-4061.2019.179382
17. Trofimov S. P. Removal of small spacecraft from the upper segment of low orbits using a sail to increase the light pressure force. Preprints of the Keldysh Institute of Applied Mathematics. 2015. No. 32. 32 pp. URL: http://library.keldysh.ru/preprint.asp?id=2015-32 (last accessed Jan. 25, 2020). (in Russian).
18. Borshcheva G. A., Maslei V. N., Shovkoplias Yu. A., Yarmolchuk E. D. Structure and key features of the SICh-2 space system. Space Technology. Missile Armaments. 2015. No. 2 (109). Pp. 16-24. (in Russian).
19. Alpatov A. P. Space Vehicle Dynamics. Kyiv: Naukova Dumka, 2016. 487 pp. (in Russian).
20. Khoroshylov S. V. Relative motion control system of spacecraft for contactless space debris removal. Science and Innovation. 2018. V. 14. No. 4. Pp. 5-8. (in Ukrainian).
https://doi.org/10.15407/scine14.04.005
21. Dmitrenko V. V., Phyo Wai Nyunt, Vlasik K. F., Grachev V. M., Grabchikov S. S., Muravyev-Smirnov S. S., Novikov A. S., Ulin S. E., Uteshev Z. M., Chernysheva I. V., Shustov A. Y. Electromagnetic shields based on multilayer film structures. Bulletin of the Lebedev Physics Institute. 2015. V. 42. No. 2. Pp. 43-47.
https://doi.org/10.3103/S1068335615020037
Copyright (©) 2020 Lapkhanov E. O.
Copyright © 2014-2020 Technical mechanics
____________________________________________________________________________________________________________________________
|
GUIDE FOR AUTHORS
====================
Open Access Policy
====================
REGULATIONS
on the ethics of publications
====================
|