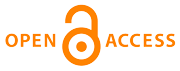 |
Головна
>
Архів
>
N 3 (2024): ТЕХНІЧНА МЕХАНІКА
>
9
________________________________________________________
УДК 629.7
Технічна механіка, 2024, 3, 124 - 138
МЕТОДИКА ФОТОГРАММЕТРИЧНОГО ВИМІРЮВАННЯ ЛЮФТІВ ТА ПРУЖНИХ ПОДАТЛИВОСТЕЙ В ШАРНІРНИХ ВУЗЛАХ ТРАНСПОРТНИХ МАНІПУЛЯТОРІВ ТА ШТАНГ КОСМІЧНИХ АПАРАТІВ
DOI:
https://doi.org/10.15407/itm2024.03.124
Бейцун В. С., Тарасов С. В.
Бейцун В. С.
Дніпровський національний університет ім. Олеся Гончара,
Інститут транспортних систем і технологій НАН України,
Україна
Тарасов С. В.
Інститут транспортних систем і технологій НАН України,
Україна
Експериментальне дослідження багатоланкових конструкцій та їх елементів є надзвичайно
актуальним і важливим для сучасної науки та техніки. Ці конструкції широко
застосовуються в багатьох галузях, зокрема в аерокосмічній, автомобільній,
робототехніці та будівництві. Наявність великої кількості з’єднаних між собою
складових робить їх поведінку складною і нелінійною. Точність і надійність таких
систем залежить від їхніх динамічних характеристик, які важко передбачити теоретично
через численні фактори, такі як податливість матеріалів, люфти в шарнірах та взаємодія
між елементами.
У даній роботі представлено розроблену та апробовану методику експериментального
визначення люфтів та пружних податливостей в шарнірних вузлах транспортних маніпуляторів
і штанг космічних апаратів, що базується на фотограмметричному методі. Проаналізовано
універсальність методу у застосуванні до різних типів механічних експериментів.
Встановлено, що отримана точність вимірювань та застосовність до різноманітних
конструкцій за неоднорідних умов робить методику в значній мірі універсальною.
При відпрацюванні розробленої методики використовувався розроблений та виготовлений
дволанковий фрагмент транспортного маніпулятора. За допомогою побудованої розрахункової
схеми для визначення впливу люфту у шарнірному з’єднанні були отримані траєкторії
розворотів секції маніпулятора з урахуваннями різних величин зазорів. Виявлено, що за
допомогою фотограмметрії можна визначати траєкторії кожного з контрольних маркерів на
шарнірі у процесі всього робочого часу вузла. Це дає змогу уточнювати розрахункові
схеми з обчисленими величинами люфтів.
Запропоновану методику можна легко адаптувати для дослідження пружних податливостей
елементів багатоланкових конструкцій у зонах локального зниження жорсткості, таких
як фланцеві з'єднання та місця кріплення допоміжного обладнання на ланках.
Показано, що розроблену методику вимірювання можна застосовувати при дослідженні
динаміки багатоланкових та наддовгих конструкцій, синхронізувавши декілька цифрових
камер. Можна реалізовувати моніторинг рухомих конструкцій у космосі дистанційно.
експериментальне моделювання, люфт у шарнірі, маніпулятор, методи візуального стеження, фотограмметрія
1. Білоус В. В. Фотограмметрія. Навчальний посібник. К.: КНУ ім. Т. Г. Шевченка, 2021. 137 с.
2. Дорожинський О. Л. Основи фотограмметрії: Підручник. Л.: Вид-во НУ «ЛП», 2003. 214 с.
3. Дорожинський О. Л., Тукай Р. Фотограмметрія: Підручник. Л.: Вид-во НУ «ЛП», 2008. 332 с.
4. Пеньков В. О. Фотограмметрія: конспект лекцій для бакалаврів спеціальності 193 – Геодезія та землеустрій. Х.: ХНУМГ ім. О. М. Бекетова, 2019. 100 с.
5. Al-Ruzouq R., Dabous S. A., Junaid M. T., Hosny F. Nondestructive deformation measurements and crack assessment of concrete structure using close-range photogrammetry. Results in Engineering. 2023. No. 18. Pp. 1–17.
https://doi.org/10.1016/j.rineng.2023.101058
6. Alemdar Z. F., Browning J., Olafsen J. Photogrammetric measurements of RC bridge column deformations. Engineering Structures. 2011. No. 33(8). Pp. 2407–2415.
https://doi.org/10.1016/j.engstruct.2011.04.015
7. Balanji H. M., Turgut A. E., Tunc L. T. A novel vision-based calibration framework for industrial robotic manipulators. Robotics and Computer-Integrated Manufacturing. 2022. No. 73. Рp. 1–15.
https://doi.org/10.1016/j.rcim.2021.102248
8. Carmo R. N. F., Valenca J., Bencardino F., Cristofaro S., Chiera D. Assessment of plastic rotation and applied load in reinforced concrete, steel and timber beams using image-based analysis. Engineering Structures. 2019. No. 198. Рp. 1–13.
https://doi.org/10.1016/j.engstruct.2019.109519
9. Chamberlain M. K., Kiefer S. H., LaPointe M., LaCorte P. On-orbit flight testing of the Roll-Out Solar Array. Acta Astronautica. 2021. No. 179. Рp. 407–414.
https://doi.org/10.1016/j.actaastro.2020.10.024
10. Dabous S. A., Al-Ruzouq R., Llort D. Three-dimensional modeling and defect quantification of existing concrete bridges based on photogrammetry and computer aided design. Ain Shams Engineering Journal. 2023. No. 14(12). Pp. 1–13.
https://doi.org/10.1016/j.asej.2023.102231
11. Dong G., Zhu Z. H. Autonomous robotic capture of non-cooperative target by adaptive extended Kalman filter based visual servo. Acta Astronautica. 2016. No. 122. Рp. 209–218.
https://doi.org/10.1016/j.actaastro.2016.02.003
12. Dong G., Zhu Z. H. Position-based visual servo control of autonomous robotic manipulators. Acta Astronautica. 2015. No. 115. Рp. 291–302.
https://doi.org/10.1016/j.actaastro.2015.05.036
13. Dong G., Zhu Z. H. Vision-based Pose and Motion Estimation of Non-cooperative Target for Space Robotic Manipulators. AIAA SPACE 2014 Conference and Exposition. (San Diego, CA, 2014). San Diego, 2014. Pp. 1–9.
https://doi.org/10.2514/6.2014-4263
14. Dong M. L., Deng W. Y., Sun Y. N., Wang Y.Q. Photogrammetric Measurement of Deformation of Large Deployable Mesh Microwave Antenna. Key Engineering Materials. 2008. No. 381. Рp. 309–312.
https://doi.org/10.4028/www.scientific.net/KEM.381-382.309
15. Goda I., L’Hostis G., Guerlain P. In-situ non-contact 3D optical deformation measurement of large capacity composite tank based on close-range photogrammetry. Optics and Lasers in Engineering. 2019. No. 119. Рp. 37–55.
https://doi.org/10.1016/j.optlaseng.2019.02.006
16. Guan X., Xu Y., Xing C. A Method for Rapid Measurement of the Deformation and Spatial Attitude of Large Radar Antennas Inside Radomes. IEEE Access. 2016. No. 4. Pp. 1–10.
17. Guo X., Yuan Y., Suo T., Su X., Liu Y., Ge Z. A novel temperature-strain synchronous measurement method. Optics and Lasers in Engineering. 2021. No. 147. Рp. 1–7.
https://doi.org/10.1016/j.optlaseng.2021.106723
18. Gwashavanhu B., Oberholster A. J., Heyns P. S. Rotating blade vibration analysis using photogrammetry and tracking laser Doppler vibrometry. Mechanical Systems and Signal Processing. 2016. No. 76. Рp. 174–186.
https://doi.org/10.1016/j.ymssp.2016.02.019
19. Iwasa T., Ota K., Harada T., Muramatsu R. High-resolution surface shape measurement of parabola antenna reflector by using grating projection method with virtual targets. Acta Astronautica. 2018. No. 153. Рp. 95–108.
https://doi.org/10.1016/j.actaastro.2018.09.031
20. Koehl M., Delacourt T., Boutry C. Image capture with synchronized multiple-cameras for extraction of accurate geometries. Int. Arch. Photogramm. Remote Sens. Spatial Inf. Sci. 2016. Vol. XLI-B1. Pp. 653–660.
doi.org/10.5194/isprs-archives-XLI-B1-653-2016
21. Lee H., Rhee H. 3-D measurement of structural vibration using digital close-range photogrammetry. Sensors and Actuators A: Physical. 2013. No. 196. Рp. 63–69.
https://doi.org/10.1016/j.sna.2013.03.010
22. Li Y., Zhang J., Han J., Cai Z., Wang Y., Li Y. Research on Flatness Rapid Measurement Technology of Large Deployable Antenna. IOP Conference Series: Materials Science and Engineering. 2020. No. 816. Рp. 1–6.
https://doi.org/10.1088/1757-899x/816/1/012022
23. Lin G. C., Bai X. H, Tan H. F., Wan Z. M. Surface Accuracy Measurement of a Novel Inflatable Antenna by Photogrammetry. Advanced Materials Research. 2011. Vol. 301-303. Рp. 713–718.
https://doi.org/10.4028/www.scientific.net/AMR.301-303.713
24. Liu T., Burner A. W., Jones T. W., Barrows D. A. Photogrammetric techniques for aerospace applications. Progress in Aerospace Sciences. 2012. No. 54. Рp. 1–58.
https://doi.org/10.1016/j.paerosci.2012.03.002
25. Liu Y., Zhang D., Hu J., Chen W., Gao C., Qiu Z. Design and structural analysis of an inflatable coated fabric manipulation arm. Thin-Walled Structures. 2019. No. 139. Рp. 310–320.
https://doi.org/10.1016/j.tws.2019.03.020
26. Luhmann T. Close range photogrammetry for industrial applications. ISPRS Journal of Photogrammetry and Remote Sensing. 2010. Vol. 65(6). Рp. 558–569.
https://doi.org/10.1016/j.isprsjprs.2010.06.003
27. Lundstrom T., Baqersad J., Niezrecki C., Avitabile P. Using High-Speed Stereophotogrammetry Techniques to Extract Shape Information from Wind Turbine/Rotor Operating Data. Conference Proceedings of the Society for Experimental Mechanics Series. 2012. Vol. 6(26). Pp. 269–275.
https://doi.org/10.1007/978-1-4614-2419-2_26
28. Ma X., Song Q., Jia Q., Fang H., Chen G. Design and experiment for a high precision reflector. IEEE International Conference on Cybernetics and Intelligent Systems (CIS) and IEEE Conference on Robotics, Automation and Mechatronics (RAM). 2017. Рp. 42–47.
https://doi.org/10.1109/ICCIS.2017.8274746
29. Ou Y., Tatsis K. E., Dertimanis V. K., Spiridonakos M. D., Chatzi, E. N. Vibration-based monitoring of a small-scale wind turbine blade under varying climate conditions. Part I: An experimental benchmark. Struct. Control. Health Monit. 2021. Vol. 28(6). Pp. 1–18.
https://doi.org/10.1002/stc.2734
30. Sabatini M., Monti R., Gasbarri P., Palmerini G. Deployable space manipulator commanded by means of visual-based guidance and navigation. Acta Astronautica. 2013. No. 83. Pp. 27–43.
https://doi.org/10.1016/j.actaastro.2012.10.015
31. Shankar N. U., Duraichelvan R., Ateequlla C. M. and etc. Photogrammetric Measurements of a 12-meter Preloaded Parabolic Dish Antenna. National Workshop on the Design of Antenna & Radar Systems (DARS), (Bangalore, 13-14 February 2009). Bangalore, 2009. Pp. 1–7.
32. Shen J., Wheeler C., O’Shea J., Ilic D. Investigation of the dynamic deflection of conveyor belts via experimental and modelling methods. Measurement. 2018. No. 127. Рp. 210–220.
https://doi.org/10.1016/j.measurement.2018.05.091
33. Sun Z., Zhang Y., Yang D. Structural design, analysis, and experimental verification of an H-style deployable mechanism for large space-borne mesh antennas. Acta Astronautica. 2021. No. 178. Рp. 481–498.
https://doi.org/10.1016/j.actaastro.2020.09.032
34. Tian Q., Flores P., Lankarani H. M. A comprehensive survey of the analytical, numerical and experimental methodologies for dynamics of multibody mechanical systems with clearance or imperfect joints. Mechanism and Machine Theory. 2018. No. 122. Pp. 1–57.
https://doi.org/10.1016/j.mechmachtheory.2017.12.002
35. Uheida K., Deng Y., Zhang H., Galuppi L., Gao J., Xie L., Mohamed A. Determining equivalent-sectional shear modulus in torsion tests for laminated glass beams using photogrammetry method. Composite Structures. 2021. No. 276. Pp. 1–14.
https://doi.org/10.1016/j.compstruct.2021.114572
36. Winstroth J., Schoen L., Ernst B., Seume J. R. Wind turbine rotor blade monitoring using digital image correlation: a comparison to aeroelastic simulations of a multi-megawatt wind turbine. Journal of Physics: Conference Series. 2014. Ser. 524.
https://doi.org/ 10.1088/1742-6596/524/1/012064
37. Winstroth J., Seume J.R. Wind turbine rotor blade monitoring using digital image correlation: 3d simulation of the experimental setup. Journal of Physics: Conference Series. 2014. Ser. 524.
https://doi.org/ 10.1088/1742-6596/524/1/012064
38. Wu T., Tang L., Du P., Liu N., Zhou Z., Qi X. Non-contact measurement method of beam vibration with laser stripe tracking based on tilt photography. Measurement. 2022. No. 187. Pp. 1–12.
https://doi.org/10.1016/j.measurement.2021.110314
39. Xiao Z., Liang J., Yu D., Asundi A. Large field-of-view deformation measurement for transmission tower based on close-range photogrammetry. Measurement. 2011. No. 44. Рp. 1705–1712.
https://doi.org/10.1016/j.measurement.2011.07.009
40. Xu Y., Guan F. Structure design and mechanical measurement of inflatable antenna. Acta Astronautica. 2012. No. 76. Рp. 13–25.
https://doi.org/10.1016/j.actaastro.2012.02.005
41. Yang J., Peng H., Zhou W., Zhang J., Wu Z. A modular approach for dynamic modeling of multisegment continuum robots. Mechanism and Machine Theory. 2021. No. 165. Рp. 1–22.
https://doi.org/10.1016/j.mechmachtheory.2021.104429
Copyright (©) 2024 Бейцун В. С., Тарасов С. В.
Copyright © 2014-2024 Технічна механіка
____________________________________________________________________________________________________________________________
|
КЕРІВНИЦТВО ДЛЯ АВТОРІВ
===================
Політика відкритого доступу
===================
ПОЛОЖЕННЯ
про етику публікацій
===================
|